Building my UV microscope has meant a steep learning curve when it comes to the useful items manufacturers have produced in the past. A really steep learning curve. The biggest issue was that the items of interest, such as objective lenses which were made with quartz or calcium fluoride elements, were often made in extremely small quantities. As such there is very little information on them, and tracking down source documents is either very difficult, or in some cases impossible. This brings me to the subject of todays post – the Leitz UV 100x NA1.20 objective. This is a high magnification objective which I was fortunate enough to obtain a copy of a few months ago, and have written about here. I keep an eye out for these and a few weeks ago found another one for sale for a reasonable amount of money, and decided to buy it as a back up copy. When it arrived, I noticed that while it looked similar to the one I already had, it was not identical. So let’s take a look at them and see what is going on with them. Here are the two objectives, my original one on the left, and the new one on the right.
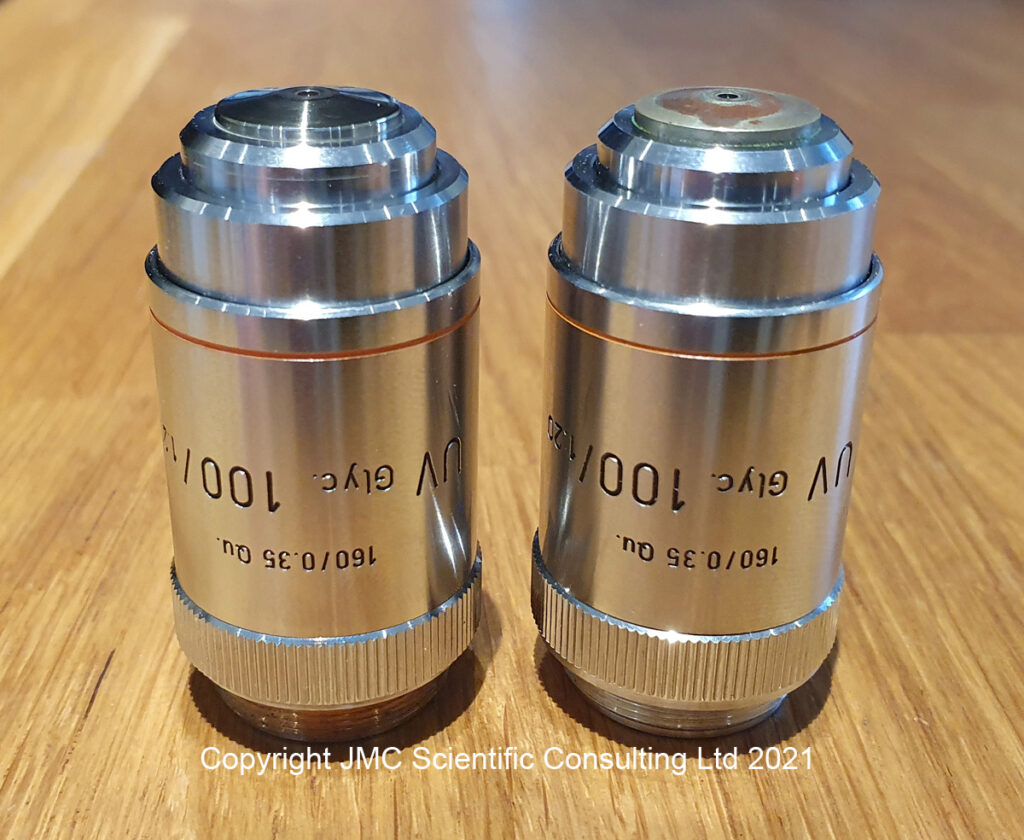
From the front, these two objective look the same as the labels are identical. The difference becomes obvious when they are turned around though.
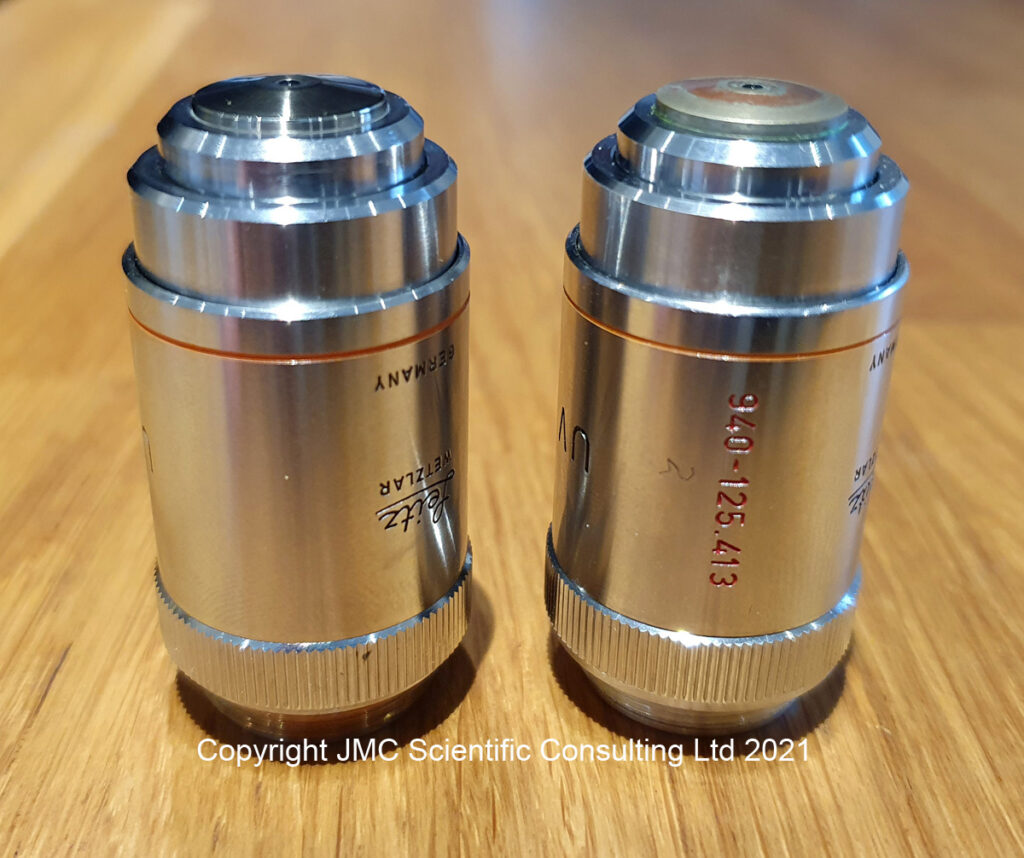
The one I got originally had “Leitz Wetzlar” and “Germany” written on the back, and that was also present on the new one. However the new one also has a 9 digit code number engraved in red on it. It also had a number “2” scratched into it. The engraving looks to be professionally done. Trying to track down the significance to this engraved number has been difficult. I’m not even 100% sure on what it is yet, but it seems to be an identification number from Leitz for a pre-production or prototype version of the objective. Interestingly I have another objective lens (a 50x phase contrast NA1.00) with a similar type of code on it, here, and I’ll come back to this one later.
How to the two compare? Some quick brightfield images of a hair on a slide of human skin, and a measuring graticule are given below (taken as a single shot through the eyepiece using a phone). Firstly, for the new one.
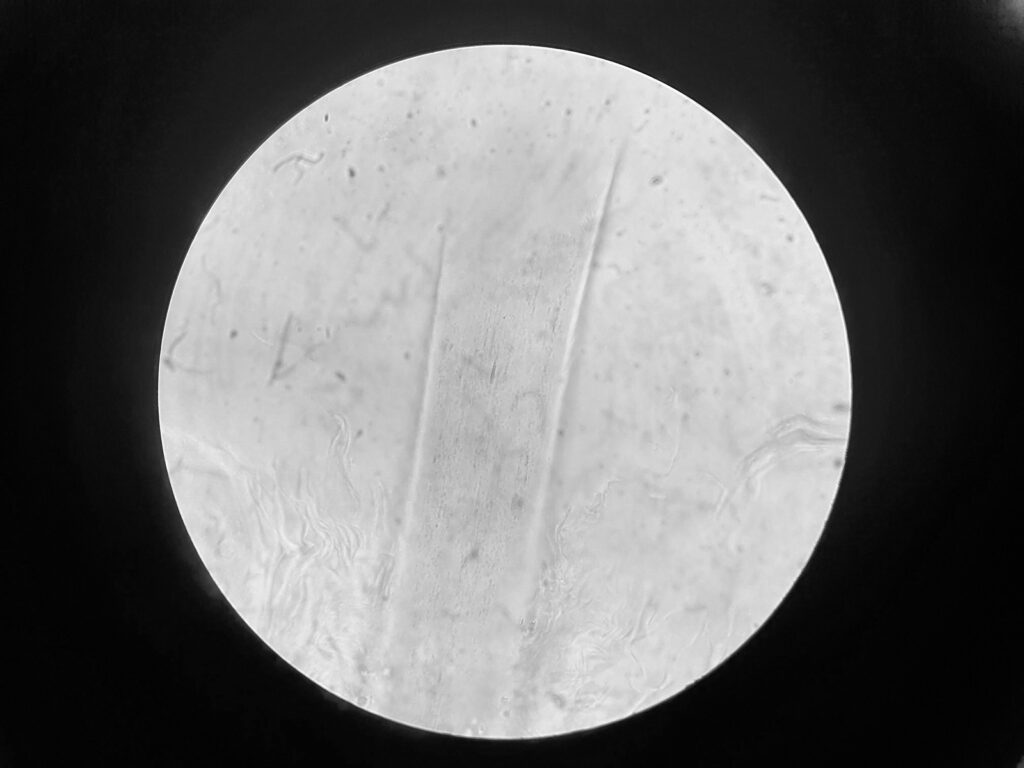
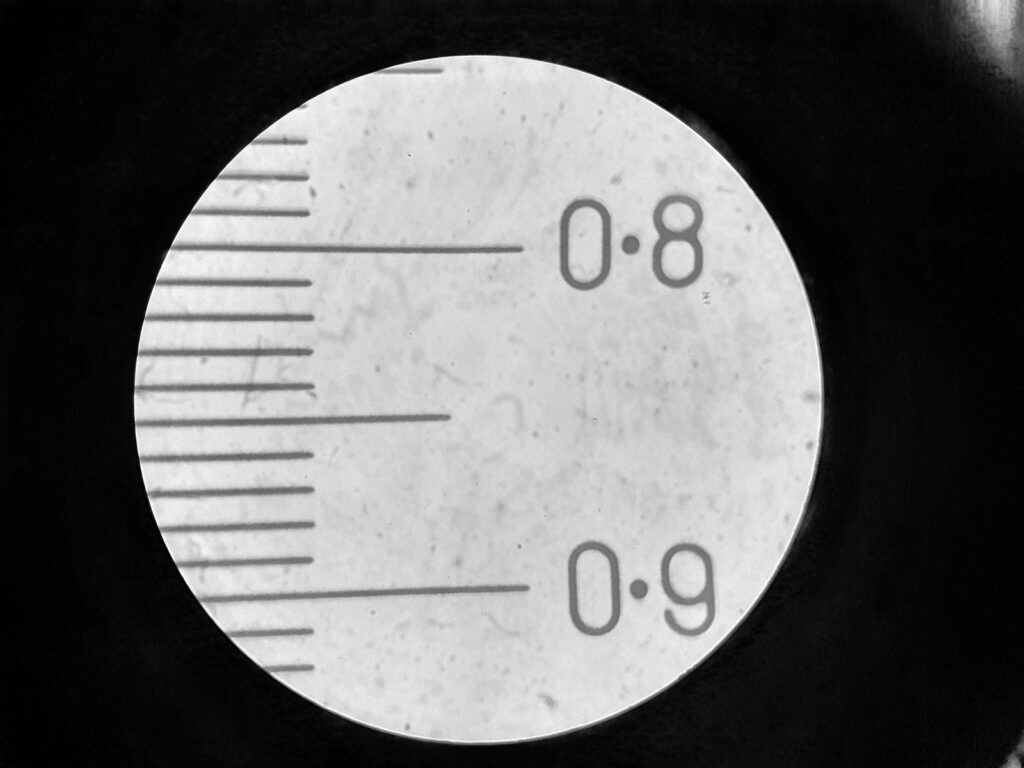
And then the other version of the lens (without the red writing on it).
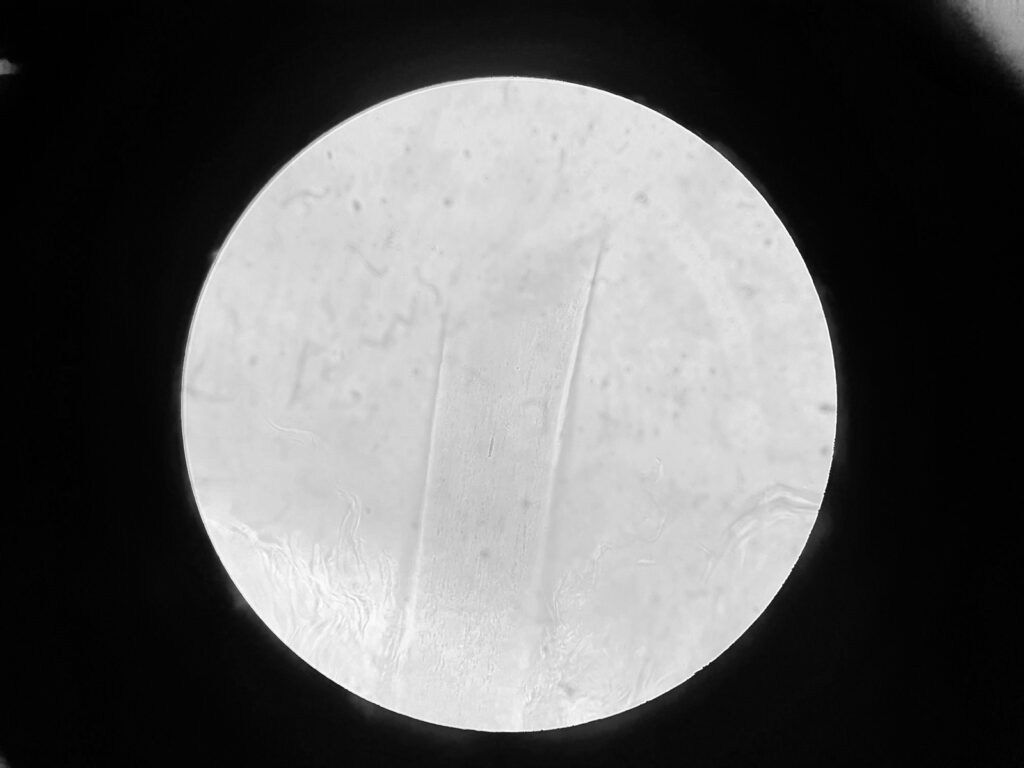
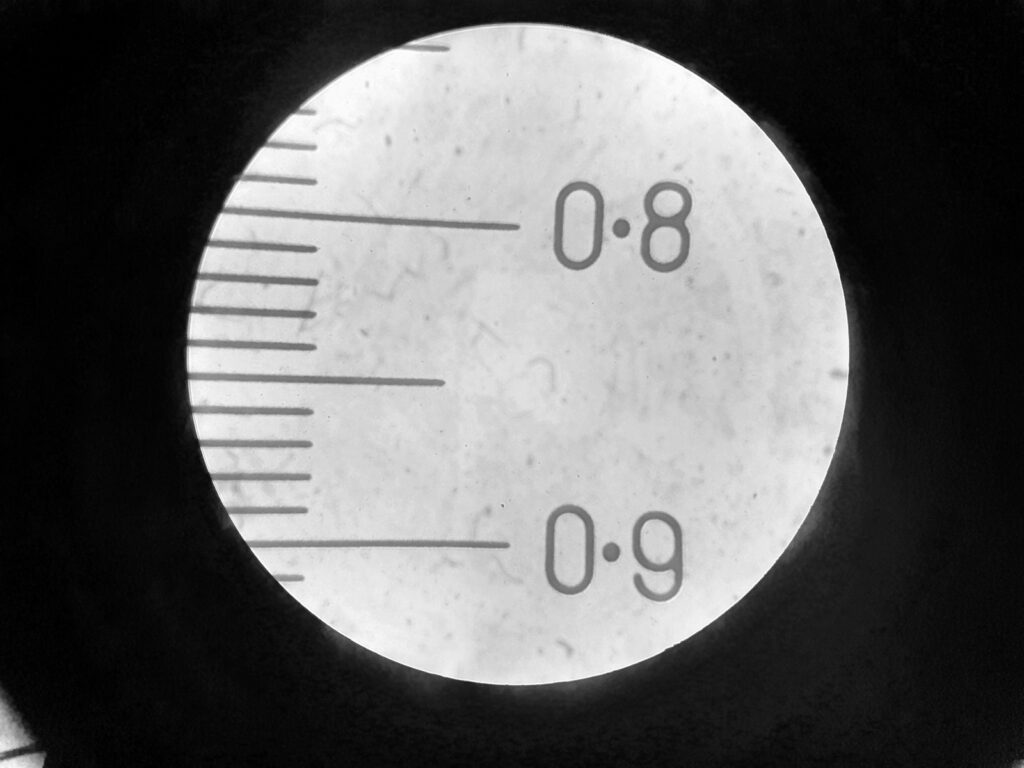
Keeping in mind that these were taken through the eyepiece with my mobile phone, there doesn’t look to be much of a difference between the two objectives. In the middle of the images of the cortex of the hair shaft, there is line of melanin granules. Cropping the original images and boosting the contrast a bit gives the following, again first for the new lens with the red writing.

And now for the other one without the red writing.

On the face of it, the two objectives look to be behaving similarly. The main reason for my interest in these is because they were designed for use with UV and to be transparent down to and below 300nm. If I compare the transmission through the two lenses, this is where something odd happens.

The original one I had transmitted down to and below 300nm as expected (note these are not absolute transmission values as the lens diameter is small and cuts off some of the beam, reducing the totoal transmission). However the one with the red writing behaves very differently to the original one. Below 400nm the transmission starts to drop until around 320nm. The slight rise again at 300nm for the one with the red numbers is likely an artifact of the measurement and not a real effect.
This is very odd and it doesn’t look as though the one with the red writing has the same transmission in the UV as the other one. Could it be that there are glass lens elements in it, instead of the UV transparent materials which it is supposed to have? Without taking them apart (which I will not be doing) it’s going to be a tough one to answer definitively.
This bring me back to something I mentioned earlier in the post. I have another Leitz lens with red writing on it – the Leitz 50x Pv lens – which I originally discussed here. When I originally tested that lens and measured the transmission I was surprised to find that it blocked the short wavelength UV even though it had ‘Quartzgl’ on the objective barrel, which I assumed meant it was made of quartz. I could not understand why this would be at the time. Could it be that these lenses with the red writing do not have the same optical elements as the final production versions? Perhaps if these are prototypes, they were mainly aimed at prototyping the overall construction rather than the specific optics which were to be used in the final production model, although the 100x ones certainly performs similarly in the visible region. That is purely speculation on my behalf, and unless I can track down an original Leitz employee who worked on them, I’ll probably never find out for certain. Although if I could find another one of the 50x Pv objectives, that would be good to test. So, anyone out there with one, feel free to get in touch.
Where does this leave us? Older equipment can be amazing for those of us involved in research and development, enabling us to buy things which would have been extremely expensive when new, for a fraction of their original price. However we should always remember that rare items such as the ones used for UV imaging were often only made in small numbers, and their suitability for the intended application needs to be verified before they can be used. Test, test and test again. If you’ve made it this far, thanks for reading, and if you’d like to know more about this or any other aspect of my work, you can reach me here.